
What is dark matter made of?
|
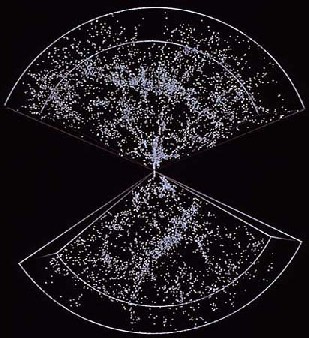 |
|
Fig. 1: Large scale structure of the Universe:
every dot represents a galaxy. Our galaxy is in the centre. The distance to the edge is about 500 million
light years .
Note the density of galaxies and large "empty spaces". The horizontal region where information is missing is that of our galaxy.
The dotted lines indicate the depth of the angular regions observed.
(Credit: Costa et al. 1994) |
The
Hubble Space Telescope
has shown that in the sky, in an angular dimension equal to that under which we see the moon, there are millions of galaxies.
With many measurements of this type it is possible to estimate that there are about 100 billion galaxies in the Universe and
that each one of these is made up on average of about 100 billion stars!
However, this enormous amount of matter is in fact not much when we compare it with
dark matter ,
whether it be "ordinary "
dark matter or "exotic".
The amount of ordinary or baryonic
(i.e. made up of protons and neutrons) matter in the Universe, whether visible or dark, can be estimated on the basis of the relative quantity of
deuterium
and helium present today and that which formed three minutes after the
Big Bang .
If there was a lot of baryonic matter at that time, collisions first between
nucleons
and afterwards between
nuclei
would have been very probable in the first moments of the Universe, and the percentage of deuterium
should now be very small because deuterium nuclei give rise to helium; if instead there was little baryonic matter,
the quantity of deuterium should be relatively greater.
From the most recent measurements of current quantities of deuterium and helium, it can be deduced that the baryonic
matter present in the Universe is only about one seventh of that needed to keep stars in their galaxies and galaxies
in their clusters. In addition, by using indirect methods, astrophysicists have estimated that there is about nine
times more baryonic matter which does not emit visible light than that which emits visible light.
What is it made of? It is enormous clouds of gas in the large
cluters
of galaxies, it is "
black holes
" arising from the collapse of massive stars and also massive black holes at the centre of the galaxies, it is "dead " stars (
white dwarfs ,
neutron stars ,
...), it is objects the size of planets, known generally as
MACHO
(MAssive Compact Halo Objects), ecc..
From what has been said so far, it is clear that the idea of the existence of non-baryonic dark matter needs to be taken seriously.
It is thought that the Universe contains a large number of
neutrinos
,
(particles which are well-known in the field of elementary particle physics), produced in the first moments of the life of the Universe.
They are rather like
"ghost particles" perché
because they do not have an electric charge and rarely interact with ordinary matter.
Precision measurements made with particle accelerators have shown the existence of three different types of neutrinos.
|
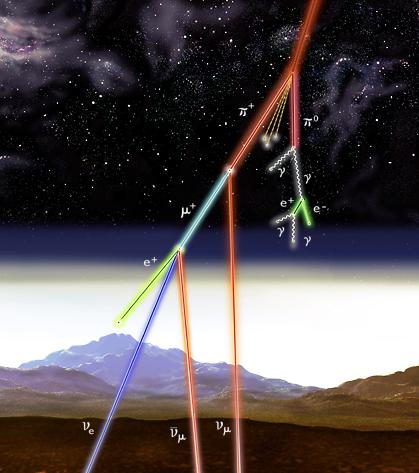 |
|
Fig. 2: Artist's impression of the production of atmospheric
neutrinos by cosmic rays
.
(Credit: INFN-Notizie
N.1 giugno 1999) |
The
Kamiokande
e
Superkamiokande
experiments in Japan,
the
Macro experiment at the Gran Sasso, and
the
Soudan 2 experiment in the United States
have presented results regarding the so-called "atmospheric neutrinos". These results strongly suggest
that a neutrino of one type can transform into a neutrino of another type (neutrino oscillations);
this can only happen if the neutrinos are not massless. The same conclusion is reached also for neutrinos coming from the sun
(the
Homestake
experiment in the USA,
GALLEX
at the Gran Sasso,
SNO in Canada, ...).
The estimated mass for neutrinos is very small, however; if we multiply it by the huge number
of neutrinos present in the Universe, we obtain a contribution to the total mass of the Universe
which is slightly less than that from visible matter. So the contribution of neutrinos with mass does not change the situation.
And their effect is reduced even further by the fact that, since they have a speed very similar to the speed of light,
they can play a role only in keeping together really enormous clusters of galaxies, not normal clusters,
and definitely not stars in a galaxy. Although it has not been possible to show this through experiments,
it is believed that their average energy is high compared in their mass, so their speed would be about the speed of light.
It is said that neutrinos form part of the
hot dark matter .
We also need to consider the possibility that, in the halos of the galaxies,
there are particles of relatively large mass which travel with a typical galactic speed of about one thousandth of the speed
of light (this is called
cold dark
matter ).
For some time now, physicists have been trying to produce such particles in high energy accelerators
but up to date they have not observed any, which could imply that they are incredibly massive; this research
will continue at future accelerators which will have much greater energy.
In addition, there are astroparticle physicists looking for such particles in
the cosmic radiation,
using sophisticated detectors where these particles should interact.
Given the rarity of these possible interactions, every type of background noise, such as cosmic rays
and environmental radioactivity, must be reduced to the minimum. Therefore the detectors must be located in underground laboratories.
But what could these mysterious, slow-moving massive particles be?
Theoretical physicists have come up with various hypotheses.
The strangest is perhaps the neutralino
which would be the
supersymmetric
electrically neutral particle with the lowest mass. The idea of the existence of super-particles (s-particles) is based on a possible
boson -
fermion ,
symmetry, which says that, corresponding to each particle with a half-integer spin (like the
electron ,
quarks ,
i
neutrinos ,
...) there must be an s-particle having a whole-integer spin (s-electron,
s-quark, s-neutrino …). In the same way, corresponding to each ordinary particle
with a whole-integer spin (eg. the
photon )
there must be an s-particle with a half-integer spin (eg. the photino).
The most credit-worthy candidate to explain cold dark matter is the neutralino,
an s-particle which can be considered as a mixture of s-particles which are
the supersymmetric partners of the photon, the boson boson
Z 0
and two Higgs bosons.
Another possibility could come from
nuclearites ,
combinations of u, d and s quarks. Ordinary atomic nuclei are formed of protons and neutrons, which are in turn formed of u and d
quarks. We would not see the equivalent of protons
and
neutrons ,
in nuclearites, but the u, d and s quarks would be free to move around inside
each nuclearite.
The nuclearites would have a higher density than ordinary nuclei and would be stable, even for masses much greater
than those of the uranium nuclei.
Another possibility could come from
magnetic monopoles ,
hypothetical particles with a magnetic charge. These can be accelerated in the galactic magnetic field
and thus would have a velocity spectrum.
There are other possible candidates: for example,
axions ,
hypothetical particles witch could remove a problem in the
Standard Model
of Elementary Particles. Axions would have a very small mass, there would be a vast amount of them,
and they would have a speed of about a thousandth of the speed of light; scientists look for them with detectors which
act as microwave receivers.
|